|
|
Types of Volcano
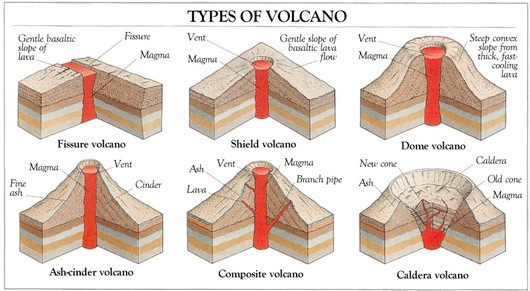
There are generally four main kinds of volcano, cinder cones, composite volcanoes, shield volcanoes, and lava domes. In addition to the four main types of volcano geologists also recognise fissure volcanoes and giant caldera volcanoes or super volcanoes. The following website provides a good description of the types of volcano.
The form of a volcano is directly linked to its location and the tectonic processes that are at work in its eruption. Both the type of eruption and the and the geographical location of the volcano are intrinsically linked to the eventual type of volcano that is produced.
Two factors are critical in the evolution of the type of volcano. Firstly, the shape of a volcano is largly determined by the viscosity of the lava it ejects. Viscosity refers to the degree of resistance to flow. High viscous lava is slow moving and quick to solidify. This generally helps build height to a volcano and helps form Composite and Dome volcanoes. Low voscosity conversely has low resistance to flow and therefore is fast flowing. Low viscous lava is more associated with low height, far reaching volcanoes such as Shield volcanoes; the largest of which can stretch as far as 130 kilometers. Viscosity of lava is determined by the quantity of silica (SiO2) in the magma. Low silica magma is called mafic and high silica magma is felsic. Silica in the magma acts to lock trapped gas bubbles in the magma. The higher the silica content the greater the gas pressure within the magma. Therefore, high silica magma produces more explosive eruptions associated with Dome and Composite eruptions. Within low silica magma the gases bubbles are freed as the magma rises to the surface. These eruption are less violent due to low pressure levels within the magma.
The form of a volcano is directly linked to its location and the tectonic processes that are at work in its eruption. Both the type of eruption and the and the geographical location of the volcano are intrinsically linked to the eventual type of volcano that is produced.
Two factors are critical in the evolution of the type of volcano. Firstly, the shape of a volcano is largly determined by the viscosity of the lava it ejects. Viscosity refers to the degree of resistance to flow. High viscous lava is slow moving and quick to solidify. This generally helps build height to a volcano and helps form Composite and Dome volcanoes. Low voscosity conversely has low resistance to flow and therefore is fast flowing. Low viscous lava is more associated with low height, far reaching volcanoes such as Shield volcanoes; the largest of which can stretch as far as 130 kilometers. Viscosity of lava is determined by the quantity of silica (SiO2) in the magma. Low silica magma is called mafic and high silica magma is felsic. Silica in the magma acts to lock trapped gas bubbles in the magma. The higher the silica content the greater the gas pressure within the magma. Therefore, high silica magma produces more explosive eruptions associated with Dome and Composite eruptions. Within low silica magma the gases bubbles are freed as the magma rises to the surface. These eruption are less violent due to low pressure levels within the magma.
The key question therefore is what determines the amount of silica in the magma?
The key factor in explaining the amount of silica in magma relates to its location. It is therfore important to relate the type of volcano and type of eruption to the plate margin on which it stands. It is well known that the most violent eruptions occur at destructive plate margins, in particular at oceanic - continental plate boundaries ,where an ocenainc plate is subducted under the continental plate. It is well known that 80 percent of the world's active volcanoes occur at the destructive margins of the 'Pacific Ring of Fire'. |
As magma rises within the lithosphere silica rich elements bond together and non- silica heavier elements begin to sink. Therefore in general terms the further that magma travels within the lithosphere and crust the more likely, heavier elements have sunk. Conversely the more likely silica rich elements have bonded. In addition, as the magma rises it assimulates with the the properties of the lithosphere and crust around it. The further up the magma travels within the lithosphere the greater concentration of silica rich elements there are to be found. therefor more and more silica is assimulated with rising heoght within the lithosphere and crust. The clear difference between plate marhins is the crucial factor. Oceanic plate is approximately 8 kilometers in depth and many places much thinner than this. Continental plates in contrast can be as deep as 70 kilometers and rareley are less than 40 kilometers.
Volcanoes and the Vocabulary
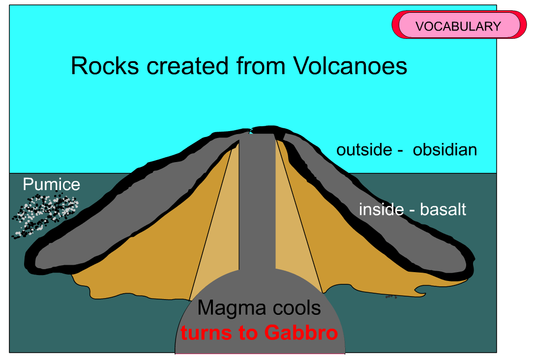
Use this animation to study the general vocabulary needed to describe the geomorphology of different volcanoes.
Types of Eruption
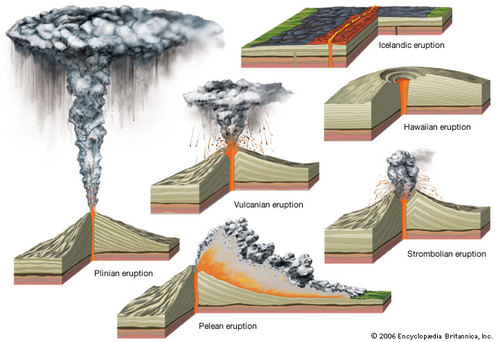
As already explained the type of eruption is strongly linked with the shape and form of the volcano. The greater the violence of the eruption correlates with the Silica (SiO2) content of the magma. Silica rich magma creates high viscosity lava that is slow moving die it great resistance to flow. This is also responsible for creating strong volcanic plugs in the crater and vents of volcanoes. This in turn increases the build up of pressure within the vent. In addition, silica rich magma restricts gas pressure within the magma and so gas pressure builds up and produces devestatingly violent eruptions. The silica content of magma is determined by the distance the magma has travelled within the lithosphere and crust. Continental crust is much deeper than oceanic crust and so we find oceanic ridges and oceanic hotspots producing shield volcanoes with Icelandic and Hawaiian type eruptions. IN contrast destructive margins where the ocenainc crust is subducted beneath the continental crust we find coposte and dome volcanoes that produce violent Vulcanian eruption and at worst Plinean and Pelean type eruptions.
The following slideshow produced by Kare Kullarud from the University of Tromso in Norway is an outstanding resource to read and extend your knowledge beyond the Post 16 level.
Minor Forms of Extrusive Activity.
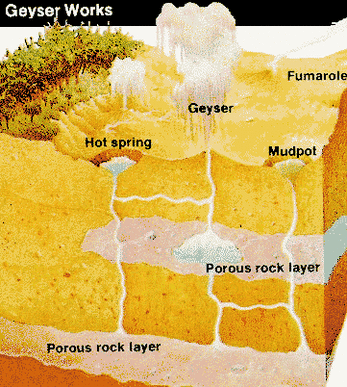
Minor forms of extrusive activity inlcude geysers, hot springs, fumaroles, sulfatara, boiling mud and mud volcanoes. This minor activity occurs in places that are geothermal and there are normally multiple examples of different activity in the same place. Geysers for example commonly occur in clusters called geyser fields.
A number of key ingredients are important to developing a geothermal region such as those found in places like Iceland, Yelowstone National Park and New Zealand. The most important ingredient include a heat source, groundwater supply, porous rock and rock structure that forms a plumbing system which contains sufficient pressure to produce superheated steam.
The diagram to the left shows a typical plumbing structure of a geyser. The surrounding rock is porous in structure and allows for easy groundwater supply. The heat source is heated rock that sits above magma that has risen to a depth of 3-5 kilometers below the surface. As water is heated by the hot rock it rises upwards within fractures in the overlying rocks. A key geologicall feature of the plumbing system is a chamber where heated water and pressure builds up. The pressure tends to be constricted by narrow vents above that are choked with sinter particles. These sinters act similar to a throttle and help build the pressure. The water above rises to surface to form a hot sprng but is cooler than the water within the chamber. Its weight above the chamber increases the pressure within the chamber. This pressure increases the boiling point and allows intense heat to develop. Eventually, the water within the chamber rapidly turns to steam erupts upwards through the hot spring above. On the surface a tall eruption of steam and water is observed. Following the eruption, a period recharge is required where water returns back into the pool and also recharges the chamber. Geysers are quite predictable and follow distinct patterns of eruption and recharge.
The video below is useful as it shows the heat source and constricted plumbing system that form a geyser
A number of key ingredients are important to developing a geothermal region such as those found in places like Iceland, Yelowstone National Park and New Zealand. The most important ingredient include a heat source, groundwater supply, porous rock and rock structure that forms a plumbing system which contains sufficient pressure to produce superheated steam.
The diagram to the left shows a typical plumbing structure of a geyser. The surrounding rock is porous in structure and allows for easy groundwater supply. The heat source is heated rock that sits above magma that has risen to a depth of 3-5 kilometers below the surface. As water is heated by the hot rock it rises upwards within fractures in the overlying rocks. A key geologicall feature of the plumbing system is a chamber where heated water and pressure builds up. The pressure tends to be constricted by narrow vents above that are choked with sinter particles. These sinters act similar to a throttle and help build the pressure. The water above rises to surface to form a hot sprng but is cooler than the water within the chamber. Its weight above the chamber increases the pressure within the chamber. This pressure increases the boiling point and allows intense heat to develop. Eventually, the water within the chamber rapidly turns to steam erupts upwards through the hot spring above. On the surface a tall eruption of steam and water is observed. Following the eruption, a period recharge is required where water returns back into the pool and also recharges the chamber. Geysers are quite predictable and follow distinct patterns of eruption and recharge.
The video below is useful as it shows the heat source and constricted plumbing system that form a geyser
Hot Springs are similar to geysers in that they require a heat source and a supply groundwater. The groundwater percolates deep into the crust and comes into conact with heated rock, which heats it making it less dense and causes it to rise. The heated water rises upwards within fractures in the rock until it pools on the surface. Some hot springs are highly gaseous and release large quantities of hydrogen sulphide.
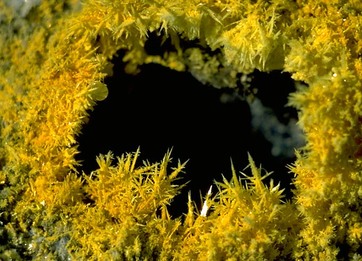
Photo: J. Alean
Fumaroles are steam and gas vents. They are very common on the flanks of active volcanoes but also found in geothermal regions, where temperatures are generally close to the boiling point of water. Solfatara is the name given to fumaroles that emit sulfurous gases.
Steaming ground occurs when steam diffuses upwards through the soil rather than following well-defined fracture within the rock. From the surface areas of steaming ground can be seen. In addition, vividly coloured rocks and soil can be found. This is the result of hot, acidic gases and fluids interacting with the rock. This interaction produces clay minerals with trace amounts of other minerals. Some of the more unusual shades include purple (from cinnabar – mercury sulfide), orange (from realgar – arsenic sulfide), and yellow to grey (from sulfur). The gases escaping from fumaroles can be rich in hydrogen sulfide. When the hot gases come into contact with the atmosphere they cool and oxidise. This process can form yellow crystals of pure sulfur around fumarole vents.
Mud pools form where steam and gas rise up to surface water ponds. The acidic gases attack surface rock and forms clay. The clay-rich soil mixes with the pond water to produce a muddy, steam-heated slurry, or mud pool. In some cases this interaction between acidic gases and rock build cone-like features or small mud volcanoes.
Steaming ground occurs when steam diffuses upwards through the soil rather than following well-defined fracture within the rock. From the surface areas of steaming ground can be seen. In addition, vividly coloured rocks and soil can be found. This is the result of hot, acidic gases and fluids interacting with the rock. This interaction produces clay minerals with trace amounts of other minerals. Some of the more unusual shades include purple (from cinnabar – mercury sulfide), orange (from realgar – arsenic sulfide), and yellow to grey (from sulfur). The gases escaping from fumaroles can be rich in hydrogen sulfide. When the hot gases come into contact with the atmosphere they cool and oxidise. This process can form yellow crystals of pure sulfur around fumarole vents.
Mud pools form where steam and gas rise up to surface water ponds. The acidic gases attack surface rock and forms clay. The clay-rich soil mixes with the pond water to produce a muddy, steam-heated slurry, or mud pool. In some cases this interaction between acidic gases and rock build cone-like features or small mud volcanoes.
Intrusive Igneous Features
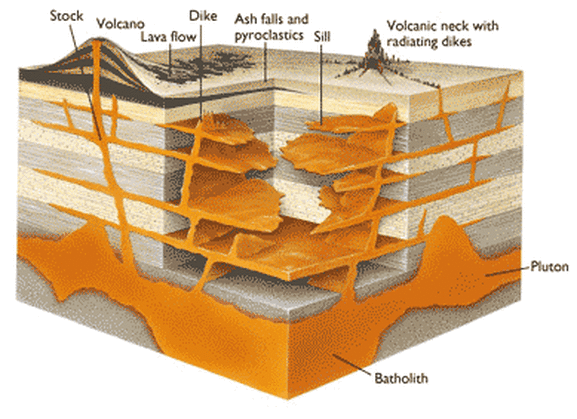
www.oldearth.org
There are many examples of volcanic features deep below the surface where magma either failed to reach the surface or became locked with in the structure of volcanoes. Overtime this magma cools and crystallizes slowly to form igneous rock called granite. The diagram to the left superbly illustrates the resulting intrusive features and associated igneous bodies. A general word used for any intrusive feature is a pluton. However a pluton is typically used to describe a large (several kilometers) non-tabular igneous feature. A magma chamber when cooled and chrystalized becomes a batholith. The batholith itself is thought to be the accumulation of several different plutons. It is the magma chamber that feeds the volcano, and sends off shoots of magma that will later crystallize into dikes and sills. Dikes are vertical intrusion that follow joints and vertical fractures within the rock. Sills are horizontal intrusions that spread out across bedding planes sedimentary layers. In some cases intense pressure builds up within a sill which causes the rock above it to rise. As the rock rises the sill pushes upwards to create a dome shape. These dome shaped sills are referred to as laccoliths. Where magma remained locked in the central vents of volcanoes, volcanic plugs have cooled and crystalized. In some places like Castle Rock in Edinburgh, these plugs have been revealed by weathering and erosion processes of the outer rock surfaces. The formation of the crag and tail feature found at Edinburgh can be seen in the diagram below. A is the crag formed from the volcanic plug, B is the tail of softer rock, and C shows the direction of ice movement that helpes erode the sedimentary rocks that once covered the plug. In the case of Edinburgh, the castle stands on the crag (A) with the Royal Mile extending along the tail (B).
Volcanic Eruption Case Studies: Nyiragongo, DRC and Etna, Italy.
Your should be able to:
- examine the nature of the volcani eruption
- discuss the impacts of the event
- comment on the management of the event
Seismicity
Seismicity refers to the frequency of earthquake activity in an area. Earthquakes follow distinct patterns of distribution that follow fault zones and reactivated fault zones. The most violent earthquakes occur at low depths associated with subductive plates at convergent margins. Transform faults and conservative margins typically produce more shallow earthquakes but with higher frequency.
An earthquake is a release of energy. They are associated with stress that builds up at faults. There are three types of stress. Compressional stress occurs at convergent plate boundaries. Tensional stress occurs at divergent plate boundaries and shear stress occurs at transform boundaries. |
When stress is released suddenly, seismic waves permeate away from the fault zone. Seismic waves can be classified into two main types, body waves and surface waves. Body waves are the fastest moving and occur in two forms, Primary waves and Secondary waves. Primary waves are fastest and travel in a longitudinal direction creating compressional stress in the direction of movement. Secondary waves are slower and transverse the direction of movement and create shear stress.
Surface waves as their name suggest move across the surface of the crust and as a consequence produce the most damage. They can be divided into two types, Love waves and Rayleigh waves. Love waves transverse across the line of direction of the seismic wave and Rayleigh waves move in a longitudinal direction in the same line of direction as the seismic wave. Love wave move objects and buildings from side to side. Rayleigh waves move objects vertically. Surface waves travel slightly slower than secondary waves.
The following resource provides an excellent overview of stresses that are generated at different fault zones. It's also interesting to see how mountains of varying scale are formed at different fault zones.
Surface waves as their name suggest move across the surface of the crust and as a consequence produce the most damage. They can be divided into two types, Love waves and Rayleigh waves. Love waves transverse across the line of direction of the seismic wave and Rayleigh waves move in a longitudinal direction in the same line of direction as the seismic wave. Love wave move objects and buildings from side to side. Rayleigh waves move objects vertically. Surface waves travel slightly slower than secondary waves.
The following resource provides an excellent overview of stresses that are generated at different fault zones. It's also interesting to see how mountains of varying scale are formed at different fault zones.
Measuring Earthquakes
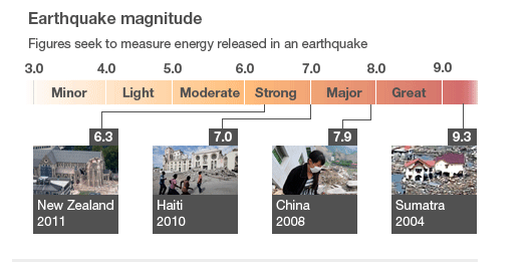
Source: BBC
The most well known form of measurement of earthquake is through the use of Seismographs and the magnitude of an earth is classified through the Richter scale. Seismographs record a zig-zag trace that shows the varying amplitude of ground oscillations (as a result of seismic waves) beneath the instrument. Sensitive seismographs, which greatly magnify these ground motions, can detect strong earthquakes from sources anywhere in the world. The time, locations, and magnitude of an earthquake can be determined from the data recorded by seismograph stations.
The magnitude of an earthquake is determined from the logarithm of the amplitude of waves recorded by seismographs. On the Richter Scale, magnitude is expressed in whole numbers and decimal fractions. For example, a magnitude 5.3 describes a moderate earthquake, whilst 7.3 denotes a major earthquake Because of the logarithmic basis of the scale, each whole number increase in magnitude represents a tenfold increase in measured amplitude. As an estimate of energy, each whole number step in the magnitude scale corresponds to the release of about 31 times more energy than the amount associated with the preceeding whole number value. Whilst the scientist is quite familiar with this scale changes of the richter scale, the layman remains quite ignorant to the differences between a 5 and a 6.
The magnitude of an earthquake is determined from the logarithm of the amplitude of waves recorded by seismographs. On the Richter Scale, magnitude is expressed in whole numbers and decimal fractions. For example, a magnitude 5.3 describes a moderate earthquake, whilst 7.3 denotes a major earthquake Because of the logarithmic basis of the scale, each whole number increase in magnitude represents a tenfold increase in measured amplitude. As an estimate of energy, each whole number step in the magnitude scale corresponds to the release of about 31 times more energy than the amount associated with the preceeding whole number value. Whilst the scientist is quite familiar with this scale changes of the richter scale, the layman remains quite ignorant to the differences between a 5 and a 6.
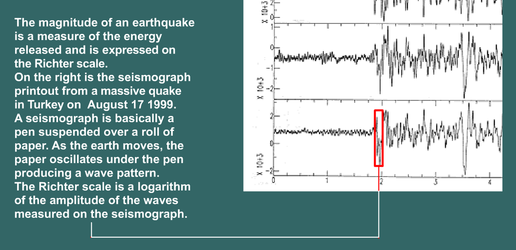
Source: Guardian
Seismologists today do not rely soley on the the Richter scale as a universal tool for measuring earthquakes, because it does not accurately measure the energy emitted in earthquakes described as Great. Instead, scientists use the moment magnitude scale, developed in the 1970s. An earthquake produces many types of waves, which radiate from its epicenter and move with a wide variety of frequencies. Compared to the Richter scale, the moment magnitude scale can account for more types of these waves, and at more frequencies. It is thus better able to estimate the total energy of earthquakes, and can also relate these observations to the physical features of a fault.
The following article from MIT News gives a very strong account of the qualities of the two types of earthquake measurement
Finally the Mercalli Scale is more of a behavioural type classification of the resulting damage caused by earhquakes. It helps draw picture of the impacts along a transect from the epicenter. The table and mapsof Alaska below show how the Mercalli can be applied on the ground. Rather than follow abstract logarithmic scale it describes the physical and human impacts of the earthquake as experienced on the ground. When mapped it becomes a very useful resource for the apllication of urban planning in regard to the mitigation of impacts for future earthquakes.
The following article from MIT News gives a very strong account of the qualities of the two types of earthquake measurement
Finally the Mercalli Scale is more of a behavioural type classification of the resulting damage caused by earhquakes. It helps draw picture of the impacts along a transect from the epicenter. The table and mapsof Alaska below show how the Mercalli can be applied on the ground. Rather than follow abstract logarithmic scale it describes the physical and human impacts of the earthquake as experienced on the ground. When mapped it becomes a very useful resource for the apllication of urban planning in regard to the mitigation of impacts for future earthquakes.
Earthquake Prediction
Firstly, it's important to state that there is no reliable scientific method for the accurate prediction of earthquakes. Long term general predictions can be made by looking at the earthquake history of an area. Recurrence intervals of major earthquakes can be usesd similar to the way flood recurrence intervals work. In this way scientists can work on the probability of an earthquake of a certain maginute occuring within a given period of time. Scientists can with some accuracy state when a minor earthquake will occur, based on their high frequency. Other faults appear to shift stress load down the fault. For example once stress has been released along one point of the fault, it begins to build up in other points further down the fault zone.
Most prediction research has been centred around the theory of Dilatancy. Dilatancy refers to the expansion or dilation of rock that takes place when it is subjected to stress. This process is caused by micro-cracks and fractures in the rock opening up and becoming larger. When a rock becomes stressed it begins to change physically. It transmits seismic waves at changing speeds, its magnetic properties can alter and its electrical resistance may also vary. The physical change in rock size maycause uplifting of the ground surface or a change in the groundwater pressure. Scientists monitor all these factors together with the hope that patterns of activity can be identified which can be associated with the build up of significant earthquakes.
A second highly disputed method relates to radon. Most rock contains small amount of gases that can be isotopically distinguished from the normal atmospheric gases. There are reports of spikes in the concentrations of such gases prior to a major earthquake; this has been attributed to release due to pre-seismic stress or fracturing of the rock. One of these gases is composed of radon, an element producecd by radioactive decay of the trace amounts of uranium present in most rock. However, extensive research into radon release produces a poor correlation with earthquake occurrence. The time lag between radon release and the earthquakes is highly variable. The spatial range is also huge.
Earthquake Case Studies: Bam, Iran and L'Aquila, Italy
Your should be able to:
- examine the nature of the volcanic eruption
- discuss the impacts of the event
- comment on the management of the event
Bam earthquake, Iran 2003
Here are a number of key resources for developing your Bam case study
BBC article - the impacts
BBC Article - the recovery
Retrospective evaluating the emergency response
BBC article - the politics
BBC article - the impacts
BBC Article - the recovery
Retrospective evaluating the emergency response
BBC article - the politics
|
|
|
|